The Base: Theoretical basis for practice schedules
Practice schedule is to perform or work repeatedly so it becomes mastered. The amount of practice a learner engages in is a critical variable, with a strong connection between the amount of practice and the amount of learning (Spittle, 2013). Practice is the most significant factor in motor skill learning. The more learning practices, the more they will learn.
Learning motor skills in a school environment can take time. Most the students don’t get enough time on each skill, to learn the skill adequately. Academic learning time in physical education (ALT-PE) is a measure of the amount of time a learner spends engaged in the learning activity (Spittle, 2013). Students can engage in motor skills practice and activities for less than 30% of the session time, with even less time in practice; less than 25% of class time in skill demonstrations and instruction; and up to 30% of the class time waiting around. (Lee & Poto 1988; Metzler 1989; Silverman 1991). Within a practice session, your aim should be to have the students engaged for as much time as possible. If we don’t plan and manage the session pedagogy, you can waste much time on management activities other than practicing skills, for example; lining up, taking the roll, collecting equipment moving places and dividing up into teams.
There are two different terms when it comes to distinguishing between types of practice schedules, Distributed and Mass, they are defined in relation to one another. In massed practice, the purpose is to maximize learning through repetition and develop motor skills. For example, when learning to play basketball, one of the first skills needed is the ability to quickly toss and catch the ball. It is important to emphasize that use of massed practice, as with any procedure used to affect learning, must take into consideration the student's current ability, limitations or restrictions in the environment. (Gillis & Romanczyk, 2005).
Massed practice includes fewer but longer sessions. The amount of rest between intervals is very short making the practice relatively continuous (Spittle, 2013). This means in a massed practice schedule, there is more work time than rest for the individuals. A massed practice schedule is beneficial to practitioners without a lot of time (Spittle, 2013). However, massed practice is considered to suppress performance as opposed to learning (Panchuk et al., 2013). As massed practice schedules involve more work than rest, this schedule negatively affects performance during practice sessions (Panchuk et al., 2013).
A Distributed practice is the same amount of practice time spread across more and shorter sessions. Usually in distributed practice there is more or equal rest time than worktime. (Spittle, 2013). Disturbed practice for example, is 2 x 1 hour sessions per week, for 8 weeks. So when kicking a ball the student should work for 10 seconds and then have a 10 second rest (Spittle, 2013). Scheduling practice sessions that are shorter and more frequent is more effective for learning than scheduling less sessions but for a longer period. For example it’s better to plan a few one hour sessions that one long four hour session (Spittle, 2013). Studies on practice sessions have show the advantage of distributed practice over massed practice. A study was completed on a sport skill by Dail and Christina (2004). Novice golfers practiced a putt of 3.7m for 240 practice trials. Learners in a massed practice schedule, practiced all their putts in one day with short breaks between each block of 10 putts. The learners in a distributed schedule, practiced 60 putts over 4 sessions, over 4 consecutive days. The distributed learners performed better than the massed learners at the end of the sessions (Dail & Christina 2004). The reason that distributed learners performed better are because of reasons such as fatigue, cognitive effort and memory consolidation. Massed practiced learners may fatigue because they aren't getting enough recovery time, The learner could also become bored and not exert as much cognitive effort (Spittle, 2013). How much effort would you put in, if you had to complete the same skill, for a long period of time with short breaks? Keeping the sessions short and sharp would reduce this possibility.
Overlearning can be defined as the ‘continuation of practice beyond the required level needed to achieve a specific performance criterion’ (Spittle, 2013). Overlearning is a tool used to reinforce and maintain the skills of the learner. The purpose of overlearning is then to not increase performance levels but rather to maintain already acquired skill levels (Spittle, 2013). Overlearning can occur immediately after the original learning experience or at later training sessions. Applying over learning at much later dates is referred to as refresher practice. Refresher practice is considered as effective as immediate overlearning (Spittle, 2013). A disadvantage of overlearning is that it is time consuming. Instructors must decide how much time in practice session should be dedicated to overlearning instead of other skills. Utilising overlearning can require additional costs to repeatedly train learners in a particular skill (Driskell, Willis, & Cooper, 1992). The concept of diminishing returns also applies to overlearning. Spittle (2013) defines diminishing returns as more practice not leading to the same level of return as the first learning experience. Reinforcing the idea that performance does not continually drastically increase with overlearning, and will begin to plateau. However, it can still enhance performance and increase proficiency (Driskell et al., 1992). It is recommended that overlearning ratios of 50 to 200 per cent are effective for retention, but also rely upon the skill being practised (Spittle, 2013). Increasing the degree of overlearning will increase the level of retention (Driskell et al., 1992).Overlearning is most effective on discrete, serial and complex skills compared to continuous and simple skills (Spittle, 2013). This is due to complex and simple skills being easier to remember compared to the others (Spittle, 2013). Without continual refresher training, retention is likely to dissipate to zero after about 5 to 6 weeks (Driskell et al., 1992).
Diminishing returns is the concept of performance continually improving with practice but at a slower rate (Spittle, 2013). During early learning stages, a rapid increase in performance can be observed (Spittle, 2013). As the athlete continues to improve, performance levels will begin to decrease and level off. This can be attributed to an individual’s genetics. Genetics are an underlying factor in determining the point of diminishing returns in an athlete (Rennie and Clark, 2006). Factors such as an individual’s muscle fibers can impact training and performance (Rennie and Clark, 2006). Training schedules applying overlearning will lead to individual’s reaching their genetic potential. Once this occurs, diminishing returns will be a factor in future training sessions.
Practice distribution can be described as the scheduling of practice sessions or the spacing of trials within a session (Spittle, 2013). The two forms of practice distribution are massed and distributed practice (Spittle, 2013). Distributed practice may be beneficial when utilized on the day of performance while massed can be beneficial in training sessions (Spittle, 2013). Massed and distributed practice schedules provide similar impacts on discrete skills (Spittle, 2013). However, learning is dependent on the number of trials attempted, and independent of the practice distributions (Panchuk, Spittle, Johnston, & Spittle, 2013). Retention of simple skills can be similar for both practice schedules, depending on the level of overlearning used by instructors (Panchuk et al., 2013). Distribution of practice should take time, overlearning and complexity of skills performed into consideration. As these factors affect performance.
The three most likely factors that can account for why distributed practice sessions are superior to massed practice sessions, in regards to performance are fatigue levels, cognitive effort and memory consolidation (Spittle, 2013). The relationship between total amount of repetitions of a skill and fatigue is a well established one. For example Sanchez-Medina and Gonzalez-Badillo (2011) where not expressly investigating the relationship between work done and fatigue level, they were investigating mechanical and metabolic response to resistance training, but in the study increased fatigue was implied when work done was increased. This relates to one of the potential explanations of the benefits of distributed practice vs massed practice. Lower fatigue levels occur in distributed practice, as it involves less total repetitions of a skill being done in each session, with longer rest periods in between. The second factor that advocates for distributed practice sessions, is that massed practice involves less cognitive effort and therefore less learning takes place. There has been research showing that partially with novice athletes, increased cognitive effort is associated with skill acquisition rate. Essentially due to the extended periods of time massed practice often goes for, it is extremely easy for the learners to become disengaged, once again especially younger or novice students/athletes. The third factor that lends weight to distributed practice sessions being superior is that it allows greater time for memory consolidation. Most of the information students/athletes remember from an individual class/training session is from the start or end of the session. As massed practice has a longer middle section of practice it is time not being used well, as time off is required to consolidate memory. Studies such as Shadmehr & Brashers-Krug (1997) showed that memory consolidation only occurs after multiple complex neuro-biochemical processes, which require time. More recent research by Walker, Brakefield, Hobson & Stickgold (2003) has even shown that sleep has an effect on memory consolidation. This is why the shorter more frequent session that distributed practice is preferable to massed practice in regards to learning, especially for less experienced athletes.
There is a general consensus that distributed practice is superior to massed practice in regards to performance but there is still debated in regards to learning. A meta-analysis by Lee & Genovese (1988) came to the conclusion that generally distributed has better learning and performance outcomes, but is more pronounced in regards to performance. They also found that the vast majority of studies on the topic where testing continuous skills. Lee & Genovese also devised distributed practice lead to increased performance and learning than massed practice, but that massed practice for discrete skills causes similar or even elevated levels of learning. This can be accounted for when considering one of factors that causes distributed practice to generally be preferable to massed practice, fatigue. As a single repetition of a discrete skill is not very physically taxing, especially when compared to a continuous, more repetitions can be performed in the same time period without as much fatigue accumulating. Essentially the debate of distributed vs massed practice, it is fairly oneside in favour of distributed. The only possible exception being is the training is for a discrete movement that does not accumulate fatigue at a great rate. Training should be brief, high intensity, to the point of the learning objective and frequent for optimal learning and performance.
Practice schedules are a crucial element in maximising practice time and effective learning. The most effective practice schedules are those that involve learners spending a large portion of the lesson engaged. Practice sessions can be distributed practice or Massed practice. Two methods that vary in length and work-to-rest ratios. Instructors choose the most appropriate method for effective learning, while also being aware of diminishing returns and overlearning.
References:
Dail, T & Christina, D 2004,’Distribution of Practice and metacognition in the learning and long term relation of a discrete motor task’, Research Quarterly for Exercise and Sport, 75, pp. 148-55.
Driskell, J. E., Willis, R. P., & Copper, C.(1992). Effect of Overlearning on Retention. Journal Of Applied Psychology, 77(5), 615-622.
Gillis, J & Romanczyk, R. In Encyclopedia of Behavior Modification and Cognitive Behavior Therapy, vol. 2: Child Clinical Applications,’Sage Publications, Thousand Oaks, CA, 2005, pp. 906-907
Lee, A & Poto, C 1988, ‘Instructional time research in physical education:
contributions and current issues’, Quest, 40, pp. 63-73.
Lee, T. D., Swinnen, S. P., & Serrien, D. J. (1994). Cognitive Effort and Motor
Learning. Quest, 46(3), 328-344. Retrieved from http://shapeamerica.tandfonline.com/doi/abs/10.1080/00336297.1994.10484130
Panchuk, D., Spittle, M., Johnston, N., & Spittle, S. (2013). Effect of practice
distribution and experience on the performance and retention of a discrete sport skill. Perceptual and Motor Skills, 116(3), 750-760.
Rennie, M., & Colson, M. (2006). Diminishing returns. New Scientist, 191(2563),
65. Retrieved from http://go.galegroup.com.ezproxy.lib.monash.edu.au/ps/i.do?p=AONE&sw=w&u=monash&v=2.1it=r&id=GALE%7CA149767383&asid=8723b76553455a68067740b0eaefd7f9
Sanchez-Medina, L., & Gonzalez-Badillo, J. J. (2011). Velocity Loss as an Indicator of Neuromuscular Fatigue during Resistance Training. Medicine and science in sports and exercise, 43(9),1725-1734. Doi: 10.1249/MSS.0b013e318213f88
Shadmehr, R., & Brashers-Krug, T. (1997). Functional Stages in the Formation of Human Long-Term Motor Memory. Journal of Neuroscience, 17(1), 409-419. Retrieved from http://www.jneurosci.org/content/17/1/409.short
Silverman, S. (1991). ‘Research on teaching in physical education’, research Quarterly for Physical Education and Sport, 62(4), pp. 352-64.
Spittle, M. (2013). Motor Learning and Skil Acquisition. 15-19 Claremont Street, South Yarra 3141, Melbourne, Victoria, Australia
Walker, M. P., Brakefield, T., Hobson, J. A., & Stickgold, R. (2003). Dissociable stages of human memory consolidation and reconsolidation. Nature, 425(1), 616-620. Retrieved from http://www.nature.com/nature/journal/v425/n6958
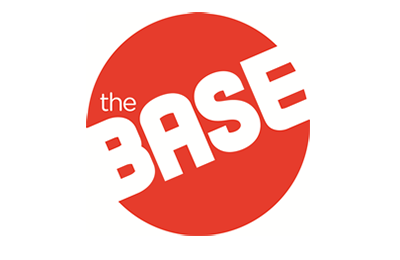
/abs/natu.html